Radiation in real time
MR-linac merges MRI technology with a linear accelerator, allowing doctors to treat tumors with greater precision
photos by Nick de la Torre
Up. Down. Sideways and around.
When people breathe, structures inside their bodies move.
"Organs, tissues and even cancer patients’ tumors move around all the time," says Geoffrey Ibbott, Ph.D., professor of Radiation Physics at MD Anderson. “It’s not unusual for a tumor to shift an inch or slightly more while a patient breathes in and out.”
In the past, this movement has confounded doctors’ ability to pinpoint a tumor’s exact location before zapping it with high-energy beams of cancer-killing radiation.
But a new device being tested at MD Anderson promises to overcome that obstacle.
The MR-linac, as it is called, merges a magnetic resonance imaging (MRI) machine and a linear accelerator into a single device. The MRI machine relays high-quality, real-time images of tumors as they’re blasted with radiation beams from the linear accelerator.
MRI machines and linear accelerators have been used in cancer therapy for years. What’s new is the two are now one.
“For the first time, this new technology lets us see where the radiation dose is being delivered, as it’s being delivered,” Ibbott explains. “The live images keep the radiation directly on target during the treatment.”
If a tumor moves out of the targeted radiation area when a patient inhales, the MR-linac automatically turns off the radiation beam. When the patient exhales and the tumor returns to its original position, radiation automatically resumes. This on-off treatment spares healthy tissue and allows doctors to deliver a more powerful dose of radiation.
“We used to treat the entire area where the tumor might travel during a normal breathing cycle,” he explains. “But with this new technology, radiation is delivered only when the tumor is located where it should be.”
Doctors watch treatment sessions on a video monitor. If what they see causes them to tweak the patient’s treatment plan, the MR-linac can refine the target and reconfigure the radiation dose in less than two minutes, all while the patient is on the table. Ibbott calls this “radiation on the fly.”
“Tailoring radiation to patients’ tumors in real time – it’s the ultimate in personalized medicine,” he says.
Scan, plan and treat
In traditional radiation therapy, doctors complete what’s called a “simulation” one week before treatment begins. The patient lies on a table in the treatment position while imaging scans identify the tumor’s exact location and characteristics. Tiny ink marks are placed on the patient’s skin to guide radiation beams during the upcoming session.
The radiation oncologist spends about a week working with medical physicists and dosimetrists who create an individualized treatment plan, including radiation dose and number of treatments based on the tumor’s size, location and type.
“All the while we’re assuming that nothing is changing during this week prior to treatment,” Ibbott says. “But in fact, things are likely changing.”
While doctors are crafting a treatment plan, the patient’s tumor may be growing, especially if it’s aggressive. Conversely, it may be shrinking if chemotherapy or other anti-tumor treatments are working. Shifts in the patient’s body weight, or fullness or emptiness in the stomach, bladder or bowels may also reposition a tumor.
“With the MR-linac, we can lock onto the tumor during radiation beam delivery,” Ibbott says, “even if it’s moving and changing shape, size or location before our eyes.”
This solves a longstanding, unmet need, he says, by allowing doctors to clearly see the tumor during treatment rather than relying on pre-treatment images.
Magnets and metal
Before the MR-linac’s debut, scientists wouldn’t dare place an MRI machine near a linear accelerator.
“That’s because MRI technology uses a powerful magnet to produce high-quality images,” Ibbott explains. “The strong magnetic field would pose an obvious safety hazard in the presence of an all-metal linear accelerator, with parts that could fly across the room at a dangerous speed toward the magnet, harming everything in their path. Also, the magnetic field would disturb the operation of the linear accelerator and degrade tumor images. Magnets and metal don’t mix.”
The MR-linac’s developers created a simple but elegant workaround to bypass this obstacle. Here’s how Ibbott explains it:
“Think of the MRI machine as a hollow paper-towel tube, with a magnetic metal coil wrapping around it. The patient slides inside the tube where imaging takes place.”
The MR-linac splits the metal coil in half, creating a gap in the middle where the patient is positioned. Radiation passes through this de-magnetized “safety zone” and images are created without distortion.
As an extra precaution, the linear accelerator portion of the MR-linac is shielded with proprietary technology that allows it to operate unaffected by the MRI machine’s magnetic field.
“These adjustments enable these two machines that previously opposed each other to now work together for the benefit of patients,” Ibbott says.
Leading the way
MD Anderson is the first clinical site in the world to install the MR-linac, other than the University Medical Center in Utrecht, The Netherlands, where it was designed with input from MD Anderson and several other institutions. Today, seven cancer centers worldwide have MR-linacs in place.
The technology has not yet been approved by the Food and Drug Administration. It’s too new, says Clifton David Fuller, M.D., Ph.D., who is teaming with Ibbott to conduct a clinical trial of the MR-linac at MD Anderson. Fuller, an associate professor of Radiation Oncology, supervises the patient treatment arm of the trial, while Ibbott directs the technical aspects including equipment installation and operation, calculating radiation dosages, and ensuring safety precautions are in place.
Data gathered at MD Anderson and other trial sites will provide the machine’s manufacturer, Elekta, with information needed to pursue FDA approval.
“Half of all cancer patients undergo radiation treatment,” Fuller says. “All could potentially benefit from this game-changing technology.”
The role of education
Medical dosimetrists work closely with the radiation oncology team — radiation oncologists, medical physicists, and radiation therapists — to create customized radiation treatment plans to target cancer while sparing normal tissue. The Program in Medical Dosimetry at MD Anderson’s School of Health Professions trains students with the professional skills of dose calculation, treatment design and quality assurance through intensive classroom and clinical education. In FY17, there were 39 students enrolled in the program, which graduated 19 this past August.

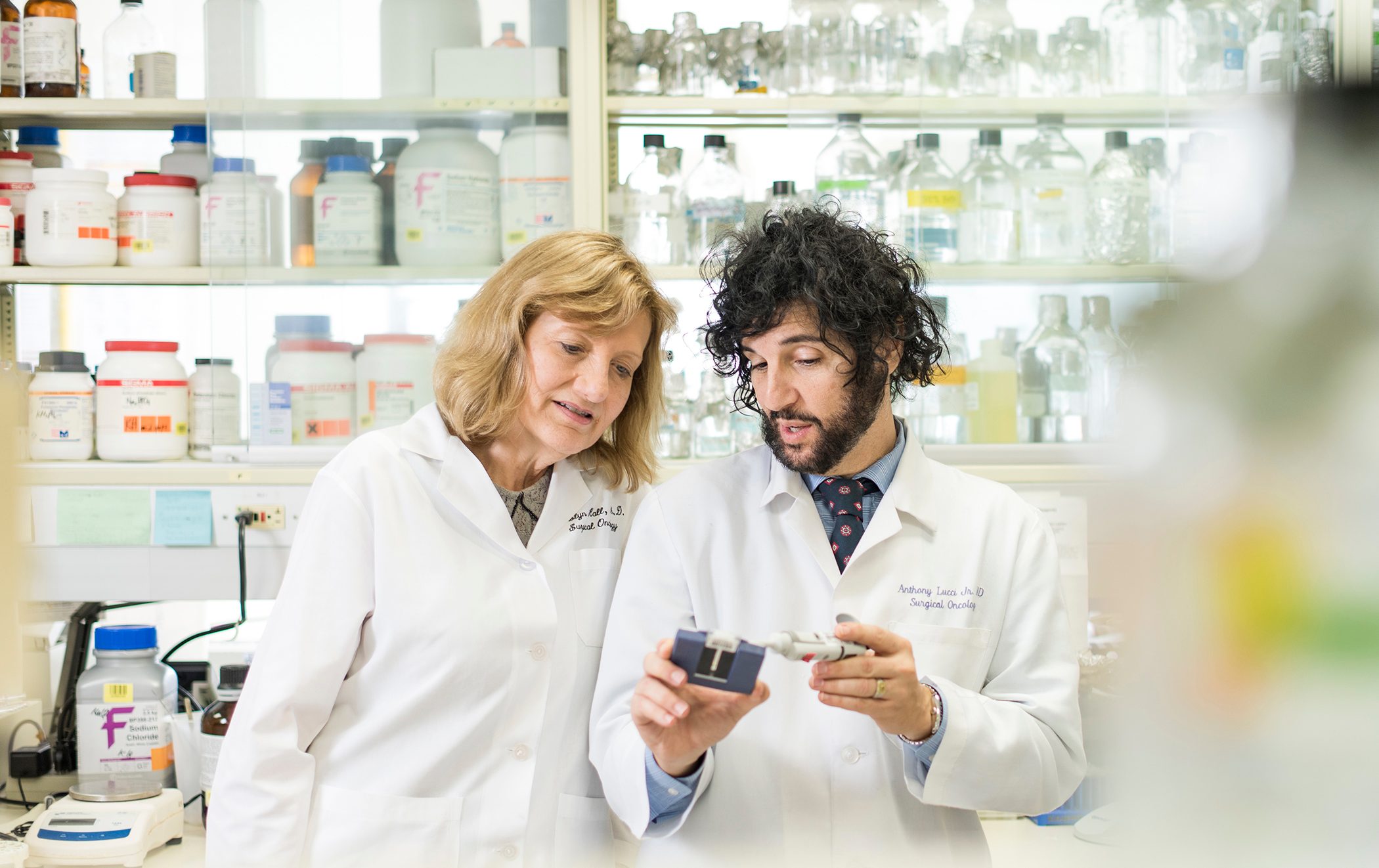
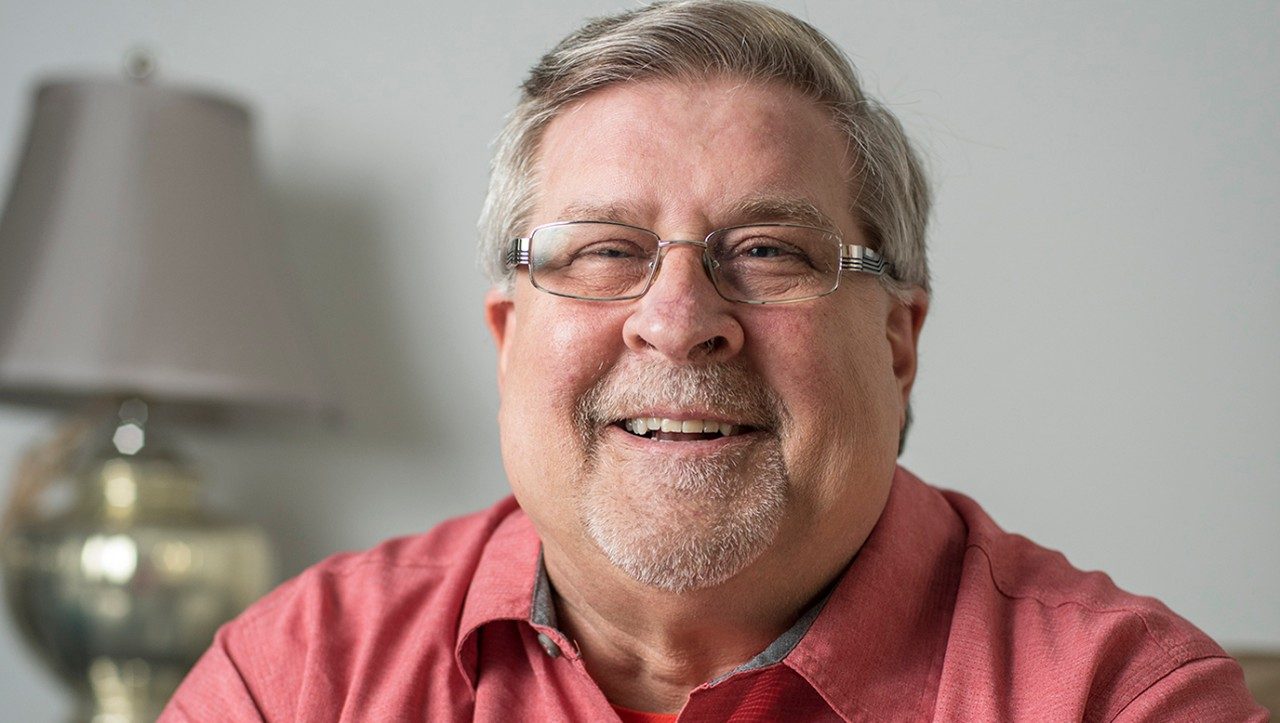




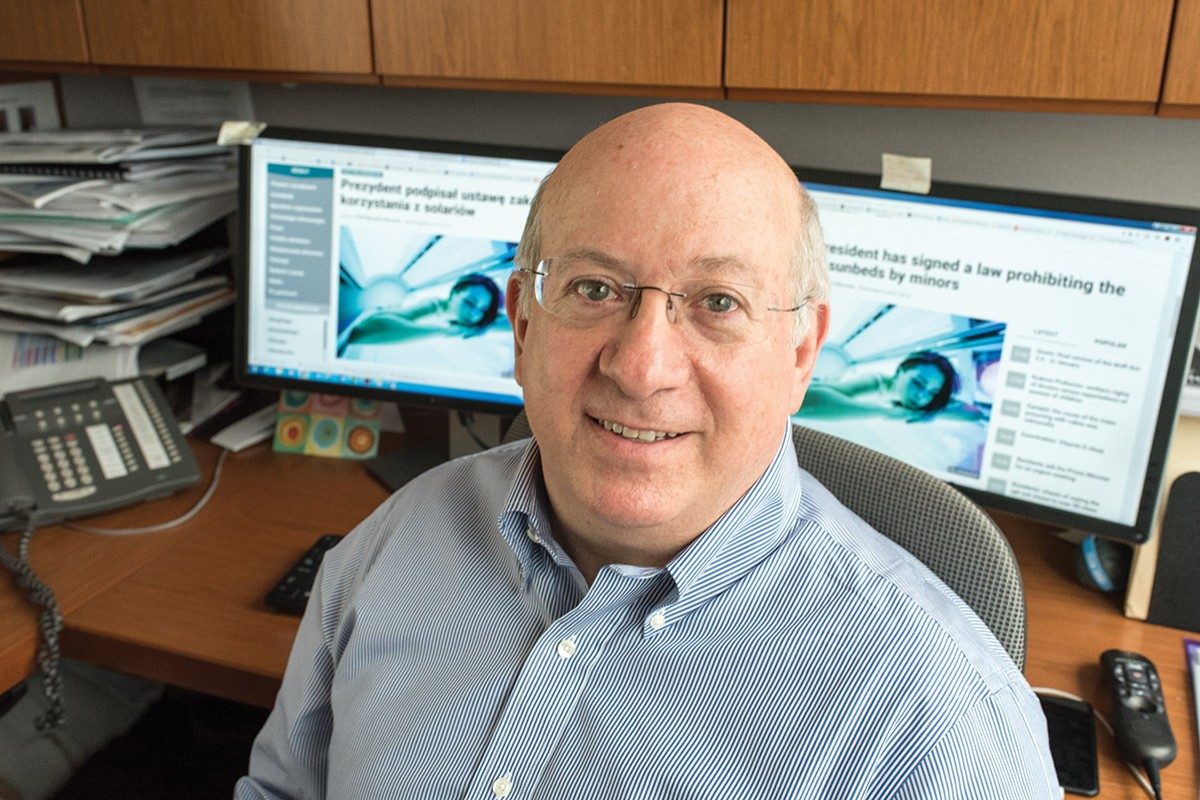
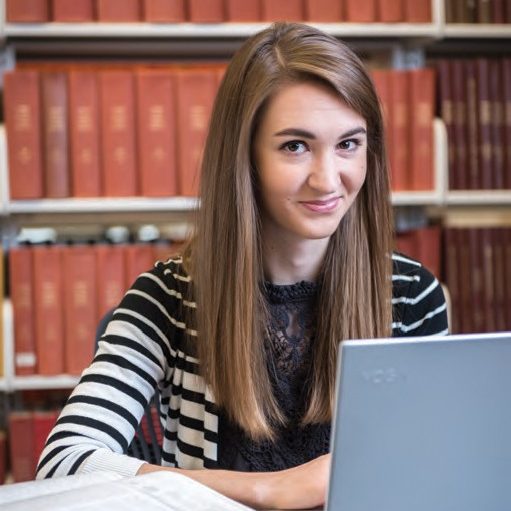
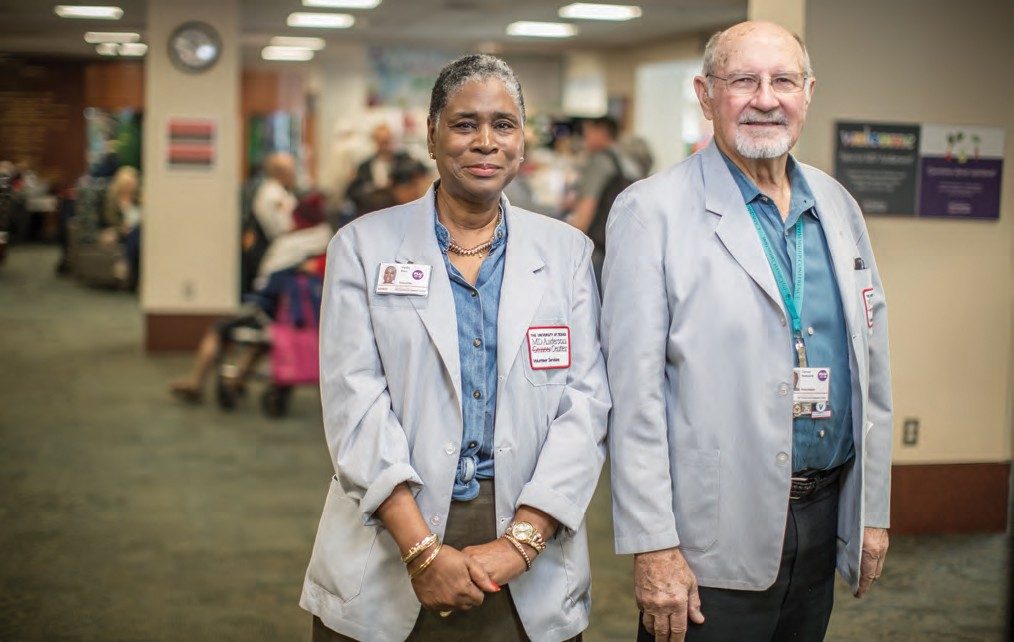